Jun Hyung Lee, M.D., Ph.D.
Endocrine Substance Analysis Center
Inductively Coupled Plasma
Mass Spectrometry (ICP-MS) is an analytical technique useful for measuring
trace elements at the μg/L or ng/L level in biological samples. This technique
is primarily used to analyze essential elements (e.g., iodine, manganese,
copper, selenium, zinc) that are measured to monitor nutritional status, and
toxic elements (e.g., arsenic, cadmium, mercury, lead) that are measured to
assess toxic effects.
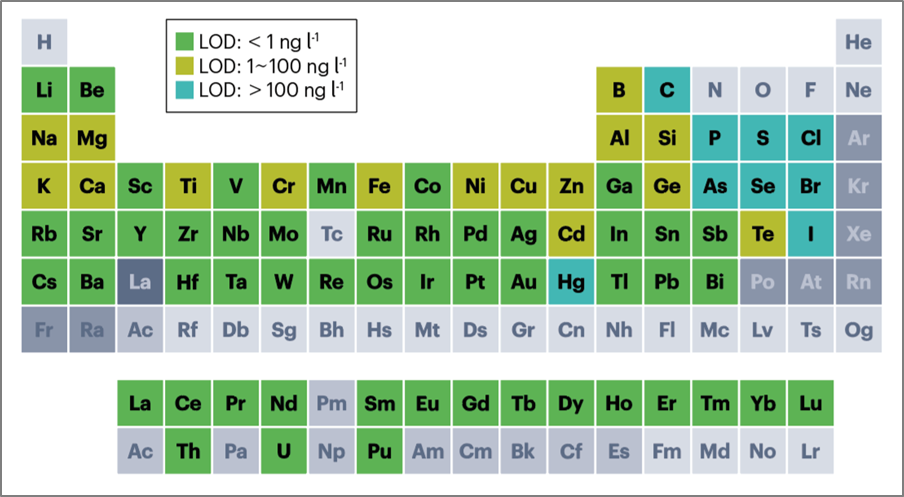
Fig. 1. Periodic table showing representative limits of detection (LOD) ranges for
Elements determined by
inductively coupled plasma mass spectrometry
Ref) Nat Rev Methods Primers. 2023;3:1–18.
Although the ICP-MS technique has been in use for over 30 years, it has only recently garnered significant interest in
clinical laboratories. One of the main advantages of ICP-MS is its ability to
perform multi-element analysis, enabling the simultaneous measurement of
several elements in a single analysis. While some laboratories still use older
techniques like Atomic Absorption Spectrometry (AAS), which can typically only
measure one or a few elements at a time, ICP-MS offers superior throughput
thanks to its ability to perform multi-element analysis, short analysis time,
and simple sample preparation, allowing it to quickly replace traditional
methods.
Technology of ICP-MS
The
ICP-MS instrument consists of six basic components: the sample introduction
system, inductively coupled plasma, interface, ion optics, mass analyzer, and
detector (Fig. 2). Liquid samples are first nebulized in the sample
introduction system to create a fine aerosol, which is then transferred to the
argon plasma. The high-temperature plasma atomizes and ionizes the sample, and
the ions are extracted through the interface region into a series of
electrostatic lenses called the ion optics. The ion optics focus and guide the
ion beam to the quadrupole mass analyzer, which separates ions based on their
mass-to-charge ratio (m/z). The ions are then measured by the detector.
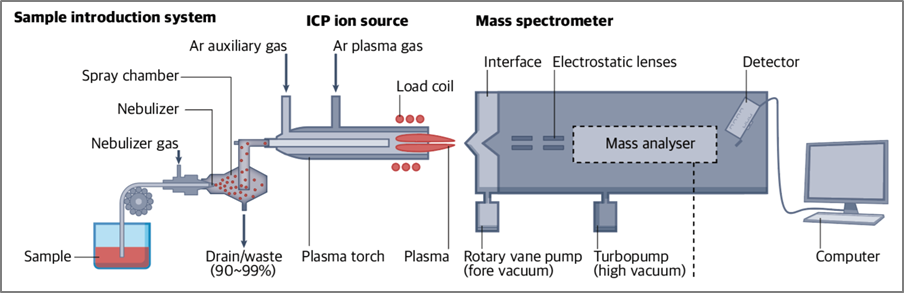
Fig.2. Structure of ICP-MS
Ref) Nat Rev Methods Primers. 2023;3:1–18
1.
Sample Preparation
Sample preparation for
ICP-MS is relatively simple. Biological samples are typically diluted or
digested with heat before analysis. Common diluents include acids (e.g., nitric
acid, hydrochloric acid) or bases (e.g., ammonium hydroxide,
tetramethylammonium hydroxide). Deionized water can also be used as a diluent,
but since some elements are unstable in pure water, acidic or basic diluents
are used in most cases.
2. Sample Induction
In
ICP-MS, liquid samples are first nebulized by a nebulizer. Various types of
nebulizers are available, including pneumatic, ultrasonic, and desolvating
types. After aerosolization by the nebulizer, the sample enters the spray
chamber, which selectively filters out larger aerosol droplets generated by the
nebulizer and stabilizes the spray pulses produced by the peristaltic pump.
3. Ionization
ICP-MS utilizes inductively
coupled plasma (ICP) for ionization. The plasma is generated by inductive
heating of argon gas with an electromagnetic coil, hence the name
"inductively coupled." This plasma generates a sufficient
concentration of ions and electrons to maintain electrical conductivity through
continuous and explosive collisions. The plasma is formed in three concentric
quartz tubes known as the torch. Argon gas flows through the innermost tube,
known as the injector, which delivers the sample aerosol to the plasma. A
concentric layer of argon gas surrounds the injector and is known as the
auxiliary gas, which helps form the plasma. The outermost layer of argon gas
serves as a coolant to prevent the torch from melting. Due to the high
temperature of the plasma, the sample aerosol undergoes desolvation,
vaporization, atomization, and ionization, transforming into ions that can be
analyzed by the mass spectrometer.
4. Mass Analyzer
The
interface separates the plasma from the mass spectrometer's vacuum chamber
through a pair of coaxial nickel (or platinum) cones. The first cone in contact
with the plasma is called the sample cone, while the second cone is called the
skimmer cone. Ions, photons, and neutral atoms or molecules are extracted from
the plasma into the interface region through a small orifice (~1 mm diameter)
at the tip of the sample cone. The ion optics guide the ion beam to the mass
analyzer and prevent photons and other neutral atoms (e.g., non-ionized matrix
components) from reaching the detector. After reaching the mass analyzer, ions
are separated based on their mass-to-charge ratio (m/z). The most commonly used
mass analyzer in clinical laboratories is the quadrupole mass analyzer.
5.
Detection
The most commonly used
detector in ICP-MS is the electron multiplier (EM). Positively charged analyte
ions strike the first dynode of the detector, which is maintained at a high
negative voltage. When ions hit the detector, multiple electrons are emitted
from the surface, and these electrons strike the next dynode, releasing more
electrons. This process of secondary emission continues, creating an
amplification cascade that ultimately generates a large enough signal to be
measured.
Quantification Methods
1. External Calibration
The number of ions hitting
the detector of an ICP-MS per second is measured in units of "counts per second" (CPS). To
convert this data into concentration values, a calibration curve is generated
using calibration standards that contain known concentrations of the element.
This method is called external calibration.
2. Internal Standardization
The use of an internal
standard allows for the correction of variations in the analytical signal due
to instrumental operating conditions or sample-specific matrix effects. A
consistent amount of internal standard is added to each sample, standard, and
blank, and results are calculated using the ratio of the analyte to internal
standard signals. The ideal internal standard has similar physical and chemical
properties to the analyte, so it behaves similarly to the analyte. Therefore,
the analyte-to-internal standard ratio should be unaffected by sample matrix or
instrumental drift. Internal standards are often integrated into the diluent
used for sample preparation (typically at 50–100 μg/L concentration) but can
also be introduced through a T-piece in the sample introduction system. The
accuracy of results obtained using this method depends on the suitability of
the chosen internal standard.
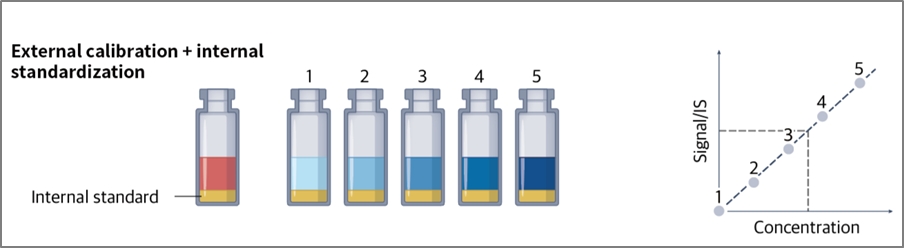
Fig. 3. External Calibration and Internal Standardization. An example of applying both external
calibration and internal standardization together to quantify the amount of a target element.
Ref) Nat Rev Methods Primers. 2023;3:1–18
Merits of ICP-MS
ICP-MS
is a preferred elemental analysis technique in various industries, including
medicine, environmental monitoring, geochemical analysis, metallurgy, and
pharmaceutical analysis, offering the following advantages:
1. High Sensitivity and Specificity
ICP-MS can typically detect
elements at concentrations ranging from 0.1 to 100 ng/L, making it highly
sensitive (Fig. 4). Its high mass resolution allows it to distinguish elements
with similar mass-to-charge ratios, providing high specificity.
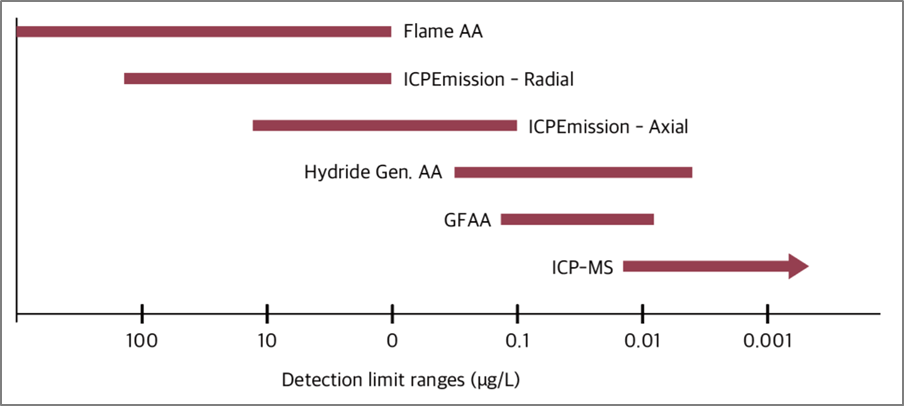
Fig. 4. Detection limit ranges of the various atomic spectroscopy techniques
Ref) Clin Biochem Rev. 2019 Aug;40(3):115–33.
2. Rapid Analysis and High Throughput
ICP-MS supports high
throughput by allowing the analysis of multiple elements in a single run,
enabling laboratories to process a large number of samples efficiently (Fig.
5).
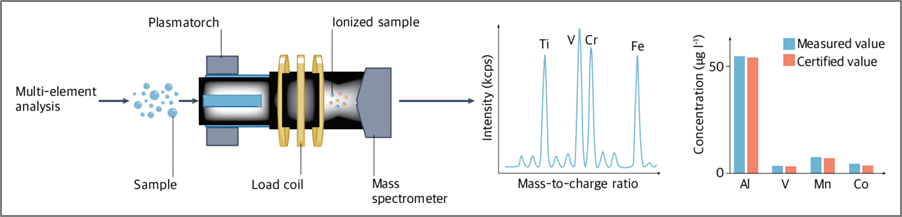
Fig. 5. Multi-element analysis function of ICP-MS
Ref) Nat Rev Methods Primers. 2023;3:1–18.
3. Wide Elemental Range
ICP-MS can analyze almost
all elements of the periodic table, providing valuable trace element analysis
results useful in clinical diagnostics (Fig. 1).
Interferences
Despite
its many advantages, ICP-MS can encounter interferences that affect its
accuracy and precision. ICP-MS interferences are classified into two types:
spectroscopic and non-spectroscopic. Spectroscopic interferences occur when
non-analyte ions share the same m/z ratio as the analyte, while
non-spectroscopic interferences are caused by sample matrix effects or
instrumental drift.
1. Spectroscopic Interference
There are four types of
spectroscopic interferences: isobaric, double-charged ion, polyatomic ion, and
tailing interference. Isobaric interference occurs when two isotopes have the
same mass within the resolution of the mass spectrometer. Double-charged
interference occurs with elements that have a second ionization potential lower
than the first ionization potential of argon. Polyatomic interference results
from the formation of polyatomic ions in the high-temperature plasma and can
occur due to incomplete atomization or recombination reactions during ion
extraction into the mass spectrometer. Tailing interference arises from overlapping
spectra of adjacent masses. To reduce or eliminate spectroscopic interference,
techniques such as sample pretreatment, matrix removal, isotope selection,
correction equations, instrument optimization, high-resolution ICP-MS, and
changes in collision or reaction gases, or tandem mass spectrometry can be
considered.
2. Non-Spectroscopic Interference
Non-spectroscopic
interferences are mainly divided into matrix effects and instrumental drift.
Matrix effects refer to the enhancement or more commonly suppression of the
analyte signal due to the sample matrix characteristics or components. These
effects arise from complex interactions of various mechanisms occurring in
almost all parts of the instrument. Methods to reduce or eliminate
non-spectroscopic interference include sample dilution, matrix-analyte
separation, and correction techniques such as internal standardization,
standard addition, and isotope dilution methods.
Clinical Applications
GC
Labs owns the largest fleet (12 units) of advanced ICP-MS equipment in Korea,
allowing it to analyze the concentrations of toxic heavy metals and trace
nutritional elements from various specimens such as blood, urine, and hair. For
example, the "13 Heavy Metals and Minerals in Blood Test" uses blood
specimens to assess exposure levels to toxic heavy metals like arsenic (As),
lead (Pb), mercury (Hg), cadmium (Cd), aluminum (Al), chromium (Cr), cobalt
(Co), and nickel (Ni), while also evaluating the status of essential trace
nutrients such as manganese (Mn), copper (Cu), zinc (Zn), selenium (Se), and
molybdenum (Mo).
Additionally,
the "40 Heavy Metals and Minerals in Hair Test" uses hair specimens,
which are relatively easy to collect, to assess exposure to heavy metals and
the nutritional status of trace elements. This test can measure elements such
as germanium (Ge), copper (Cu), sodium (Na), lead (Pb), nickel (Ni), rubidium
(Rb), lithium (Li), magnesium (Mg), manganese (Mn), molybdenum (Mo), vanadium
(V), barium (Ba), platinum (Pt), beryllium (Be), boron (B), arsenic (As),
bismuth (Bi), selenium (Se), mercury (Hg), strontium (Sr), zinc (Zn), antimony
(Sb), aluminum (Al), iodine (I), uranium (U), silver (Ag), phosphorus (P), tin
(Sn), zirconium (Zr), iron (Fe), cadmium (Cd), potassium (K), calcium (Ca),
cobalt (Co), chromium (Cr), thallium (Tl), tungsten (W), thorium (Th), titanium
(Ti), sulfur (S), and others, in a single analysis.
Conclusion
ICP-MS is a highly
sensitive analytical technique capable of measuring trace elements in a wide
range of clinical specimens. It is particularly suitable for clinical testing
due to its high sensitivity, ability to measure multiple elements
simultaneously, excellent throughput, and simple sample preparation. While
ICP-MS is a highly specific and sensitive method, operators must be aware of
potential interferences and the analytical factors that may affect the accuracy
of reported results. A properly set up and managed ICP-MS system can provide
highly sensitive and accurate test results down to ng/L levels.
Reference
01. Van Acker T, Theiner
S, Bolea-Fernandez E, Vanhaecke F, Koellensperger G. Inductively coupled plasma
mass spectrometry. Nat Rev Methods Primers. 2023;3:1–18.
02. Wilschefski SC and
Baxter MR. Inductively Coupled Plasma Mass Spectrometry: Introduction to
Analytical Aspects. Clin Biochem Rev. 2019 Aug;40(3):115–33.
03. McPherson RA, Pincus
MR, editors. Henry’s clinical diagnosis and management by laboratory methods.
24th ed. Philadelphia: Elsevier; 2021